The Color of Life: Unveiling Blood Chemistry
Written on
Chapter 1: Introduction to Blood Chemistry
Let’s delve into the fascinating world of blood composition. Blood is a complex mixture comprising various components such as plasma, red blood cells, white blood cells, and platelets, all of which you might recall from your middle school science classes. However, its most notable feature is undoubtedly the striking red hue. As I began to explore this topic, two key questions piqued my curiosity: 1) What is the chemical basis for this vivid red color? and 2) How does blood transport oxygen?
While I won't delve too deeply into the chemistry to avoid overwhelming anyone with technical terms, it’s essential to touch on these queries. Chemistry, particularly organic chemistry, has its own intricate language, which I aim to simplify. Nevertheless, these questions provide a segue into some intriguing facts about chemistry and its broader implications.
Hemoglobin and Porphyrins
Many attribute the red color of blood primarily to hemoglobin, which can be a bit misleading yet partially accurate. Hemoglobin is a protein found in red blood cells, and it contains a specific arrangement of atoms known as a “heme group.” This heme group is largely responsible for the blood's color. Take a look at the structure of hemoglobin shown below.
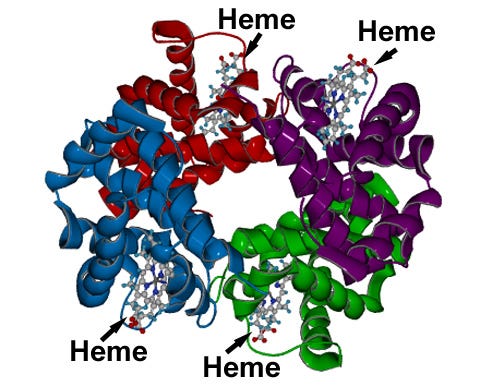
The representation above illustrates hemoglobin, where different colors indicate various sections—it's a single molecule.
If the colorful depiction seems daunting, focus on the fact that heme groups are fundamental components of hemoglobin. Within a red blood cell, the heme group belongs to a broader class of molecules called porphyrins (pronounced “por-fer-ins”), which are known for their vivid colors in various applications. Before examining where we encounter porphyrins, let’s briefly explore their structure.
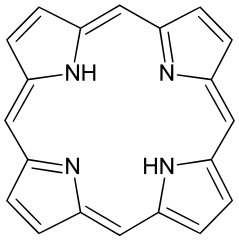
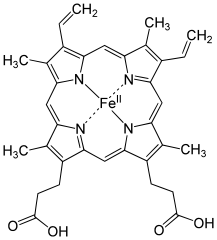
To clarify, “porphyrin” refers both to a specific molecule and a class of molecules sharing a similar structure. The comparison between porphyrin and Heme B highlights their resemblance. Heme B, being more intricate, gives rise to the red color we associate with blood. Two primary factors contribute to the color of Heme B: 1) the various groups attached to it (like “CH3,” “O,” “OH,” etc.) and 2) the presence of “Fe^II” at its core.
To clarify, “Fe^II” indicates iron, with the “II” signifying its oxidation state. This detail is somewhat tangential; the key takeaway is the presence of iron. If copper were to replace iron, the blood would appear blue, akin to the blood of octopuses and other mollusks, which use copper instead of iron in their porphyrins.
Understanding this can be challenging, as we often associate copper with its familiar reddish-brown appearance. However, when copper loses electrons, it adopts a completely different hue, a phenomenon applicable to many metals.
Moreover, copper isn't the only metal that can occupy the center of a porphyrin. Nature has experimented with various elements, resulting in fascinating combinations. For instance, cobalt in the center yields vitamin B12, while magnesium produces chlorophyll. These are just examples of naturally occurring porphyrins; laboratory-synthesized variants can include metals like palladium, platinum, and zinc. These synthesized compounds often serve as catalysts or are investigated for pharmaceutical applications, including cancer treatments. My personal favorite synthetic porphyrin is copper phthalocyanine (pronounced “thal-oh-cyah-neen”), better known in the art world as "phthalo blue." If you've heard of phthalo blue, you might share my fondness for the renowned painter Bob Ross.
The Chemistry of Color | Artrageous with Nate explores the relationship between chemistry and color, offering insights into how pigments are formed and their significance.
An Unusual Bond in Heme B
In typical chemical structures, solid lines connect atomic symbols to indicate bonds. However, in the case of Heme B, you'll notice a dashed line between Fe (iron) and N (nitrogen). This is intentional and signifies a different kind of bond known as a “dative” or “coordinate” covalent bond. Unlike regular covalent bonds, where electrons are shared between two atoms, a dative bond involves both electrons coming from one atom, making it less robust.
Understanding chemical bonds can be simplified: they represent connections between atoms. The strength of these bonds reflects the affinity between the atoms involved. While chemists use numerical values to measure bond strength, the fundamental concept remains the same—chemical bonds are associations that meet a certain threshold of strength.
Returning to dative bonds: the one in Heme B formed by iron and nitrogen (the dashed line) is similar to the bond formed when oxygen attaches to iron. These bonds are roughly one-tenth as strong as a typical carbon-hydrogen bond, which is often used as a reference for measuring bond strength in organic chemistry.
Interestingly, the precise mechanism of how oxygen binds to heme groups is a topic of ongoing research and remains somewhat elusive. In an era where cancer gene analyses can be completed in under a day thanks to advanced computing power, it's intriguing that we still lack definitive answers regarding oxygen's binding mechanism to hemoglobin. This complexity of nature is both humbling and fascinating.
Fortunately, this complexity facilitates the straightforward transfer of oxygen. If oxygen transfer required breaking a bond, our lives could be drastically different. Instead, the transfer resembles a simple exchange of a business card—it's not bound by anything, allowing it to move freely.
At this point, we’ve explored the connection between blood color, Bob Ross, and the unique chemistry of oxygen binding. This exploration has unveiled the science behind blood's color and introduced a new type of chemical bond.
As a closing note, I’d like to express my gratitude to reader Geoff for inspiring this article with his suggestion in the comments. If you have any topics you'd like to see discussed, please feel free to share your ideas!
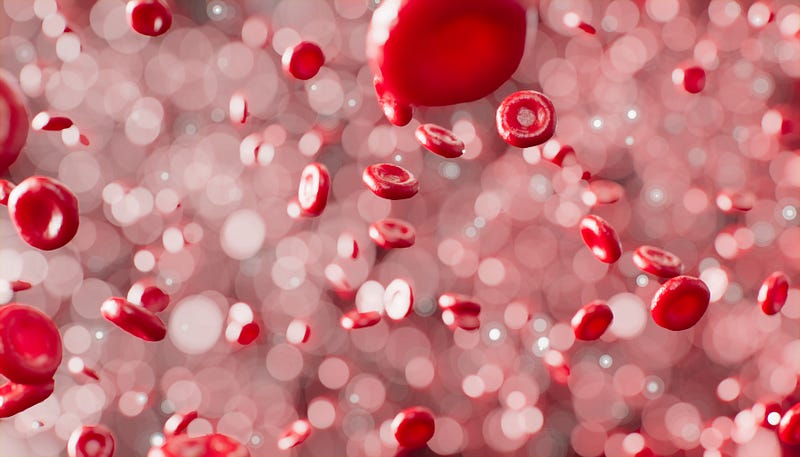
Appendix — Additional Insights
For those interested, the sources I consulted regarding the strength of oxygen's association with hemoglobin are quite technical. Nonetheless, they consistently highlight the complexity of studying this process.